
Gregor Mendel's Work in Genetics: The Laws of Inheritance
The study of genetics, the science of heredity, owes much of its foundation to the pioneering work of Gregor Mendel, an Austrian monk who lived in the 19th century. Mendel's experiments with pea plants uncovered the fundamental principles of heredity, leading to the formulation of Mendel's Laws of Inheritance.
Mendel's Experiments
Gregor Mendel's journey into the world of genetics began with his meticulous and systematic experiments with pea plants, Pisum sativum, carried out in the mid-1800s. Mendel's choice of pea plants as a model organism was particularly astute because they have easily distinguishable traits and a relatively short reproductive cycle, making them ideal for controlled experimentation.
Selection of Traits:
Mendel selected seven distinct traits to study, including seed shape, seed color, flower color, and flower position. These traits exhibited clear and easily distinguishable variations.
Purebred Parental Plants:
To initiate his experiments, Mendel started with true-breeding or purebred parental pea plants. These plants consistently exhibited a particular trait, either dominant or recessive, with each generation. For example, he used purebred tall plants and purebred short plants for the trait of plant height.
Cross-Pollination:
Mendel meticulously performed controlled cross-pollination. He removed the anthers (male parts) from one plant to prevent self-fertilization and then transferred pollen from the anthers of another plant with a different trait. This process ensured that Mendel could precisely control the mating of pea plants and study the inheritance of specific traits.
First Filial (F1) Generation:
The results of Mendel's initial crosses were astonishing. When he crossed purebred tall plants with purebred short plants, the offspring of the first filial generation (F1) were all tall. This outcome contradicted the prevailing belief that blending inheritance was the mechanism of heredity. Instead, Mendel observed that the traits of the parents remained distinct in the F1 generation.
Self-Fertilization of F1 Generation:
Mendel allowed the F1 generation plants to self-fertilize by using their own pollen. This self-fertilization led to the creation of the Second Filial (F2) generation.
Second Filial (F2) Generation:
The F2 generation proved to be even more intriguing. In this generation, Mendel observed a 3:1 ratio of tall to short plants. This result was consistent across multiple traits, supporting Mendel's conclusion that there was a precise mechanism governing the inheritance of traits.
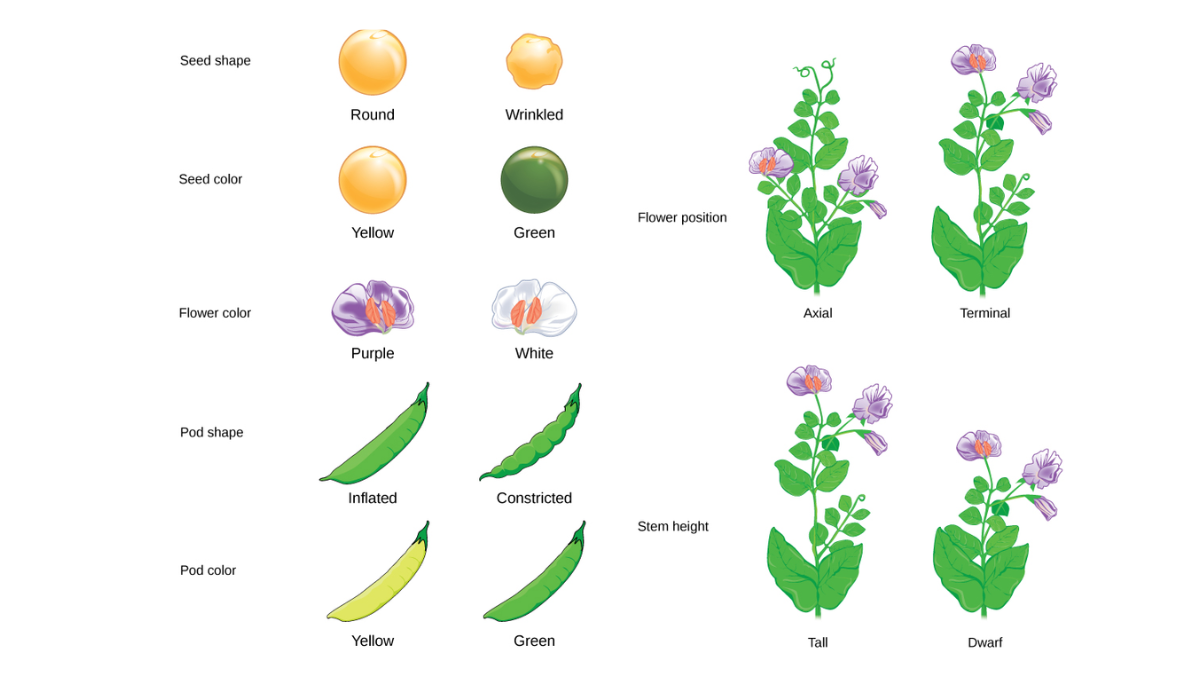
Law of Segregation
Mendel's first law, the Law of Segregation, is a fundamental principle of heredity that explains how alleles segregate or separate during the formation of gametes (sperm and egg cells). This law can be summarized as follows:
Each individual possesses two alleles for a particular trait, one inherited from each parent. These alleles segregate during gamete formation, ensuring that each gamete carries only one allele for a given trait.
Mendel's experiments with pea plants beautifully illustrate the Law of Segregation. When he crossed purebred tall (TT) and short (tt) plants, the F1 generation was composed of all tall plants, each with one "T" allele from one parent and one "t" allele from the other parent. These F1 plants produced gametes with either a "T" or "t" allele, ensuring that when they self-fertilized in the F2 generation, the alleles segregated independently, resulting in the 3:1 ratio of tall to short plants.
The Law of Segregation is a cornerstone of genetics and helps us understand how genetic diversity is maintained in populations. It explains why offspring inherit a combination of traits from their parents and how traits can reappear in subsequent generations, even if they were not visible in the immediate offspring.
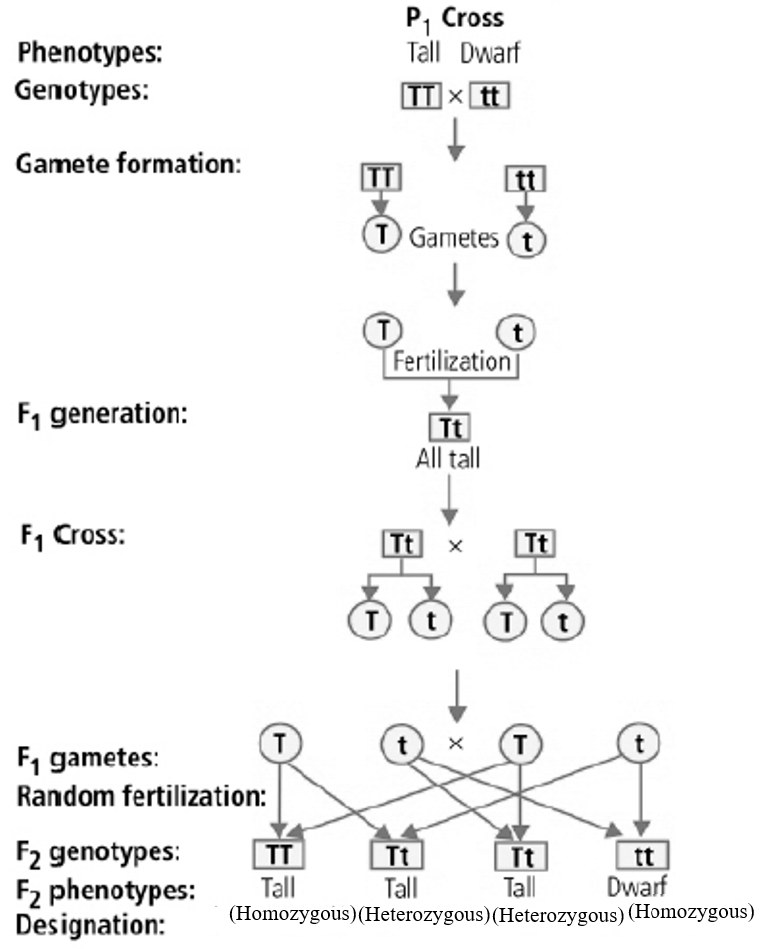
Law of Independent Assortment
Mendel's second law, the Law of Independent Assortment, builds on the foundation laid by the Law of Segregation.
This law states that the inheritance of one trait is independent of the inheritance of another trait. In other words, the alleles for different traits assort independently during gamete formation.
To illustrate the Law of Independent Assortment, Mendel examined two different traits simultaneously, such as seed shape (round or wrinkled) and seed color (yellow or green). He crossed purebred parental plants with one dominant and one recessive allele for each trait (e.g., RRYY for round and yellow, rryy for wrinkled and green).
Mendel's observations revealed that the traits of seed shape and seed color segregated independently in the F2 generation. This independence allowed for various combinations of traits, as seen in the F2 offspring, supporting the Law of Independent Assortment.
The Law of Independent Assortment has significant implications for understanding genetic diversity. It explains why offspring can inherit a wide range of trait combinations, leading to the genetic variability that is crucial for adaptation and evolution in populations.

Conclusion
Gregor Mendel's groundbreaking experiments with pea plants laid the foundation for the field of genetics. His work led to the formulation of two fundamental laws of inheritance: the Law of Segregation and the Law of Independent Assortment. These laws provided a systematic and accurate framework for understanding how traits are passed from one generation to the next.
Mendel's discoveries revolutionized our understanding of heredity, moving us away from the idea of blending inheritance and toward the concept of discrete hereditary units, which we now call genes. His work, although initially overlooked, has since become the cornerstone of genetics and remains instrumental in our ongoing exploration of the genetic mechanisms that shape life on Earth.
Mendel's Laws continue to be relevant in the modern field of genetics, providing a solid foundation for understanding the inheritance of traits and the complexities of genetic diversity. By appreciating Mendel's contributions, we can better comprehend the intricate tapestry of life's inheritance patterns and the vital role genetics plays in the diversity and adaptation of living organisms.